Lay Summary
PGAP2-CDG, also known as hyperphosphatasia with mental retardation syndrome 3 (HPMRS3), or Mabry syndrome, is a rare inherited neurological disorder. There have been 18 cases of PGAP2-CDG reported to date in the medical literature. PGAP2-CDG is classified as a disorder of GPI anchor biosynthesis and is caused when an individual has mutations in both copies of the PGAP2 gene. PGAP2 provides instructions for making a protein that participates in building GPI anchors which are molecules that “anchor” certain proteins to the cell surface. The PGAP2 protein functions in one of the final steps of GPI anchor biosynthesis which involves modifying the lipid component of the GPI anchor which is necessary for the attachment of GPI-anchored proteins to the cell membrane. Mutations in PGAP2 cause GPI-anchored proteins to be unstable or unable to attach to the cell surface. Symptoms of PGAP2-CDG begin in infancy and are primarily characterized by intellectual disability, seizures, reduced muscle tone, and developmental delay. Screening tests are available for PGAP2-CDG, but a definitive diagnosis can only be achieved through genetic testing. There are currently no approved treatments for PGAP2-CDG, and treatment is focused on the management of specific symptoms and complications.
Overview
Post-GPI Attachment to Proteins 2 Congenital Disorder of Glycosylation (PGAP2-CDG) is a rare autosomal recessive disorder. The PGAP2 gene encodes a protein that is involved in the lipid remodelling step of the GPI anchor biosynthesis in the Golgi where PGAP2 transfers a saturated fatty acid chain to the GPI anchor. Fatty acid remodeling of the GPI anchor helps stabilize the attachment of GPI-anchored proteins to the lipid-rich cell membrane. GPI-anchored proteins play an important role in the body, and mutations in PGAP2 results in these proteins being released from the cell membrane.
The first reported case of PGAP2-CDG was in 2013, and there have been 18 cases reported to date1–6. Symptom onset begins in infancy and the characteristic presentations of patients with PGAP2-CDG include intellectual disability, hypotonia, seizures, and developmental delay1–6. Facial dysmorphism may also be present in patients with PGAP2-CDG. A definitive diagnosis can only be acquired through genetic sequencing, however, analyzing blood cells by flow cytometry to detect an absence of cell surface GPI-anchored can assist in the screening for disorders of GPI-anchor biosynthesis. There are currently no approved therapies for PGAP2-CDG, and management is based on responding to individualized patient needs.
Synonyms
- Hyperphosphatasia with Mental Retardation Syndrome 3 (HPMRS2)
- GPI biosynthesis defect type 8 (GPIBD8)
- Mabry Syndrome
Inheritance
PGAP2-CDG is an autosomal recessive disorder, meaning an affected individual inherits one defective copy of the gene from each asymptomatic patient.
Gene Function
The PGAP2 gene encodes a transmembrane protein that participates in the lipid remodelling of GPI-anchored proteins in the Golgi. PGAP2 catalyzes the addition of the saturated fatty acid called stearic acid to the lipid segment of the GPI anchor, thereby replacing the unsaturated fatty acid that was removed by PGAP7,8. Lipid remodelling is critical for GPI-anchored proteins to associate in lipid rafts in the plasma membrane. Lipid rafts are domains within the plasma membrane that are enriched in glycosphingolipids, cholesterol, and GPI-anchored proteins and regulate different cellular processes, such as signalling transduction9.
GPI-Anchor Protein Biosynthesis
GPI-anchored protein biosynthesis is one of the major glycosylation pathways that attach glycans to lipid molecules within cells. Many proteins are attached to the cell surface by GPI anchors, which are referred to as GPI-anchored proteins.
The core structure of GPI consists of phosphatidylinositol (PI), glucosamine (GlcN), three mannose sugars (Man3), and phosphoethanolamine (EtN-P) connected to each other in that sequence (Figure 1). GPI-anchored proteins are attached to the GPI by forming a bond between the EtN-P group of the GPI core and the C-terminus of newly synthesized proteins in the ER lumen10,11.

Figure 1. Overview of GPI-anchored protein structure.
The GPI anchor is added to proteins, generating GPI-anchored proteins. The GPI anchor section lodges into the membrane, attaching the protein to the membrane. The GPI anchor involves phosphatidylinositol, glucosamine, mannose and phosphoethanolamine.
The generation of GPI-anchored proteins is a multi-step process involving more than 30 enzymes and can be divided into the following steps:
-
GPI anchor synthesis
-
Protein attachment
-
Lipid/glycan remodelling and transport of the GPI-AP
GPI anchor synthesis (step 1) is largely carried out by a series of enzymes encoded by the PIG genes, while enzymes encoded by the PGAP genes facilitate the remodelling of the GPI-AP (step 3).
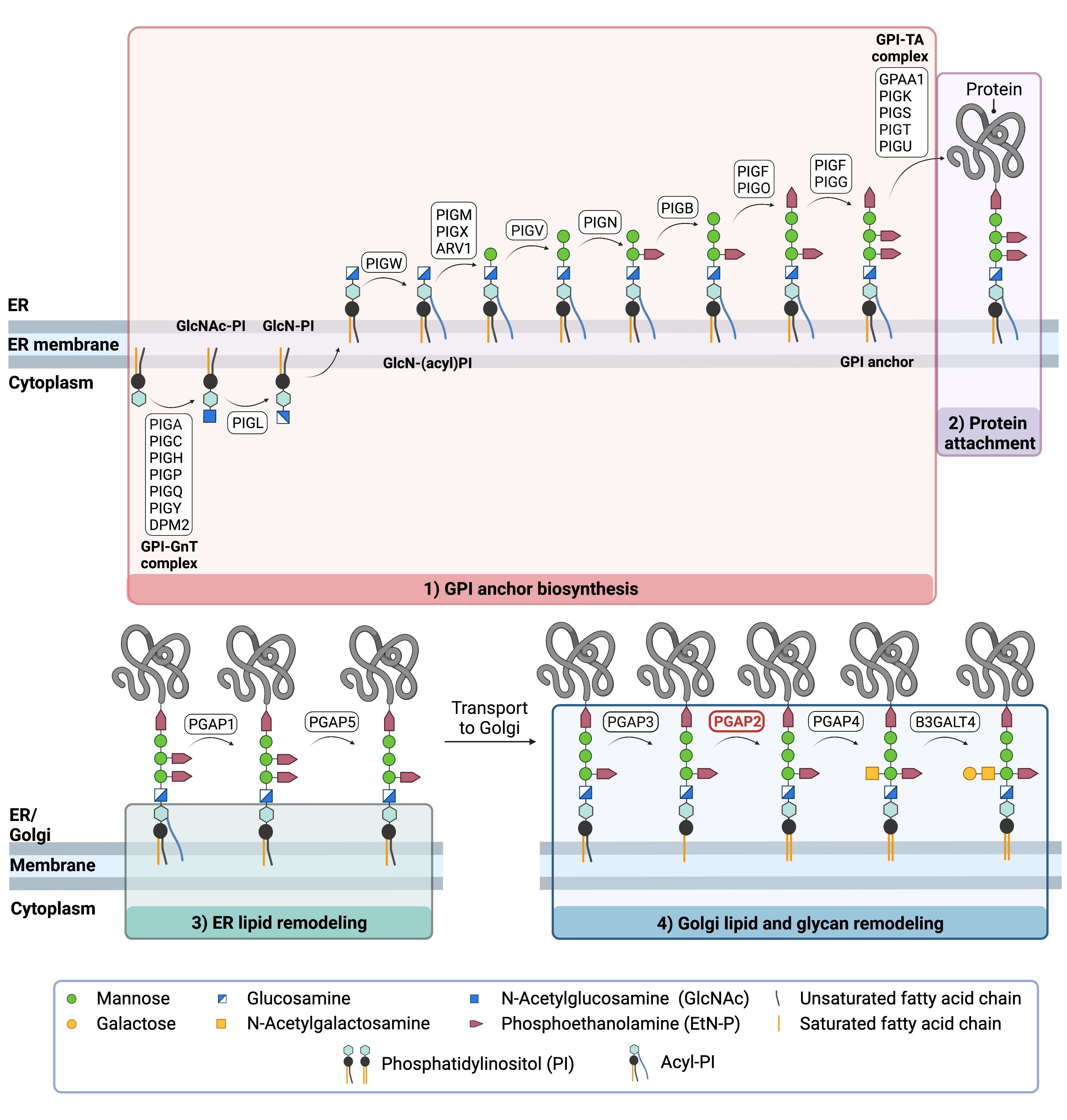
Figure 2.Overview of GPI-anchored protein biosynthesis and role of PGAP2.
GPI-anchored protein biosynthesis involves a series of enzymatic reactions. First, the GPI anchor core is built on the ER membrane, where enzymes modify the lipid and glycan portions, generating PI-GlcN-Man3-EtN-P. The protein is then attached to the GPI anchor in the ER, before further modifications are made to the lipid and glycan portions in the ER and the Golgi. In the Golgi, PGAP2 catalyzes the addition of the saturated fatty acid to the GPI anchor, thereby replacing the unsaturated fatty acid that was removed by PGAP3.
GPI Anchor Synthesis
The first stage of GPI anchor biosynthesis involves the stepwise construction of the GPI anchor. N-acetylglucosamine (GlcNAc) is added to the lipid phosphatidylinositol (PI), generating GlcNAc-PI. GlcNAc-PI then has its acetyl (Ac) group removed from the GlcNAc sugar, generating glucosamine phosphatidyl inositol (GlcN-PI) which is transported into the luminal side of the ER10,11.
Within the ER, a fatty acid chain is attached to GlcN-PI. The final steps of the GPI anchor synthesis involve the sequential addition of 3-4 mannose sugars and three EtN-P groups by several PIG enzymes. The mannose residues are transferred from the ER-resident sugar donor, dolichol-phosphate mannose. The completed GPI anchor core, PI-GlcN-Man3-EtN-P, is then ready to be attached to a protein10,11.
Protein Attachment
Once the GPI anchor has been synthesized, it is transferred en bloc to a protein with a C-terminal GPI attachment signal sequence by the GPI transamidase (GPI-TA) complex. This complex catalyzes the simultaneous cleavage of the signal sequence and attachment of the GPI anchor to the newly synthesized protein10,11.
Lipid/Glycan Remodelling and Protein Transport
After the protein has been attached to the GPI anchor, both the glycan and lipid portion of the anchor undergo modifications (referred to as remodelling) in the ER and Golgi by post-GPI attachment to protein (PGAP) enzymes. In the ER, lipid remodelling includes the removal of the acyl chain and one EtN-P group, which enables the protein to be transported to the Golgi. In the Golgi, the fatty acid chains of the anchor are exchanged with other lipids that better enable the protein to associate in lipid rafts in the plasma membrane 7,10–12.
In the first step of lipid remodelling in the Golgi, PGAP3 catalyzes the removal of the unsaturated fatty acid chain. In the second step, a saturated fatty acid chain is re-added by PGAP2 8. Once fatty acid remodelling is completed, some GPI-anchored proteins are modified by the addition of an N-acetylgalactosamine (GalNAc) glycan. The mature GPI anchored protein is then transported to the plasma membrane where it self-associates in lipid raft7,10–12.
Disease Mechanism
Mutations to the PGAP2 gene lead to partially reduced levels of the PGAP2 protein. This results in a decrease in functional GPI-anchored proteins, as PGAP2 is required for their stable association within lipid rafts in the cell membrane. As a result, GPI- anchored proteins are instead secreted from the cells once they reach the membrane at the end of the secretory pathway. Since GPI-anchored proteins are required for neural development, as well as cellular signal transduction 9, mutations in the PGAP2 gene lead to various impairments throughout the body6.
Mutations
The PGAP2 gene is located on chromosome 11 (11p15.4). To date, 10 disease-causing variants in the PGAP2 gene have been reported; all of which are missense variants1–6. Notable mutations include the c.191C>T (p.Ala64Val) variant, which was the only reported mutation to cause microcephaly in a patient4. Another patient with the c.221G>A (p.Arg74His) mutation had a particularly severe phenotype, with gastrostomy used for feeding, and tracheostomy and mechanical ventilation being used5.
Signs & Symptoms
Clinical Presentation
Individuals with PGAP2-CDG typically develop signs and symptoms during infancy. PGAP2-CDG is primarily characterized by severe global developmental delay, epilepsy, and hypotonia. Symptoms of PGAP2-CDG include11–6:
- Neurological – global developmental delay, intellectual disability, low muscle tone (hypotonia), epileptic seizures, brain abnormalities, absent speech
- Gastrointestinal - feeding problems, Hirschsprung disease
Additional symptoms of PGAP2-CDG may include a small head size (microcephaly), facial dysmorphism (short nose with broad nasal bridge, small jaw, tented upper lip), congenital heart defects and sensorineural hearing loss.
Biochemical Abnormalities
Elevated levels of alkaline phosphatase in the blood (hyperphosphatasia) are often observed in individuals with PGAP2-CDG.
Classification
PGAP2-CDG is classified as a disorder of GPI-anchor biosynthesis.
Diagnosis
GPI-related CDG should be considered in individuals presenting with early onset severe seizure disorders and dysmorphic facial features, even if transferrin and total N-glycan analysis are normal. As currently available screening tests for CDG will not reliably detect PGAP2-CDG, diagnosis is typically achieved through genetic testing, either as part of an epilepsy panel or whole exome sequencing. PGAP2-CDG may also be primarily screened for by analyzing surface GPI-anchor proteins on blood cells by flow cytometry.
GPI-Anchored Protein Flow Cytometry
Individuals with PGAP2-CDG show an absence of GPI-anchored proteins on the surface of granulocytes, a type of white blood cell.
Biomarkers
No biomarkers for PGAP2-CDG have been reported.
Prognosis
The prognosis of PGAP2-CDG may vary depending on the severity of an individual’s symptoms. As of 2016, the oldest reported patient was 64 years of age and had the c.881 C>T (p.Thr294Met) mutation2.
Management
Management of symptoms may include combinations of physical therapy, occupational therapy, and palliative measures. A ketogenic diet has been used to manage treatment-resistant epilepsy, and gastrostomy and tracheostomy combined with artificial ventilation have been used to treat feeding and breathing problems, respectively5.
Therapies
There are currently no therapies available for PGAP2-CDG.
Research Models
Research models are available to study PGAP2-CDG, including yeast and mouse models.
Yeast (S. cerevisiae)
Cwh43 is the human PGAP2 homologue in yeast. Cwh43 knockout yeast display an absence of ceramide-containing GPI-anchors in their cell walls, as this gene is responsible for remodelling the fatty acid ceramide on GPI-APs13.
Mouse (M. musculus)
Constitutive Pgap2-/- mouse
Homozygous Pgap2-/- mice are embryonically lethal before E9.0 (IMPC)9.
Pgap2Clpex/Clpex mouse
In a forward genetic ENU mutagenesis screen, a Clpex (cleft lip and palate, edema, and exencephaly) mutant mouse was identified114. Homozygous Clpex mutants were determined to have a hypomorphic homozygous missense mutation in the initiating methionine c.A1G (p.M1V) in exon 3 of Pgap2. Pgap2Clpex/Clpex mice survived to E18.5 and embryos displayed multiple partially penetrant phenotypes including cranial neural tube defects, defects in frontal bone ossification and decreased limb length9.
Pgap2clpex/null mouse
Pgap2clpex/null mice have both a missense and (p.M1V) and a null allele at the Pgap2 locus. Mutant mice survive to E13.5- E14.5 and display neural tube defects, reductions in limb length, reduced heart muscle thickness, and sometimes cleft lip as well as a cleft palate9.
Chinese Hamster Ovary Cell Line
Chinese Hamster Ovary (CHO) Pgap2 knockout cells secrete GPI-anchored proteins CD55 and CD59 into the medium3,7.
Human Cell Lines
Human Embryonic Kidney (HEK) Cells
A HEK293 knockout library of genes involved in GPI biosynthesis in HEK293 cells was generated for functional studies15. Knockout of PGAP2 in HEK293 cells results in a reduced expression of CD55 and CD599,15.
Clinical Studies
Active
Clinical and Basic Investigations into Congenital Disorders of Glycosylation (NCT04199000)
The Frontiers in Congenital Disorder of Glycosylation Disorders Consortium (FCDGC) is conducting a 5-year natural history study on all CDG types, including PGAP2-CDG. The purpose of this study is to define the natural history and clinical symptoms of CDG, develop new diagnostic techniques, identify clinical biomarkers that can be used in future clinical trials and evaluate whether dietary treatments improve clinical symptoms and quality of life.
Publications
PGAP2-CDG Scientific Articles on PubMed
Additional Resources
OMIM
IEMbase
Orphanet
Genetic Testing Registry
ClinVar
NIH
Marrvel
GeneCards
UniProt
References
- Perez, Y. et al. A Rare Variant in PGAP2 Causes Autosomal Recessive Hyperphosphatasia with Mental Retardation Syndrome, with a Mild Phenotype in Heterozygous Carriers. Biomed Res. Int. 2017, (2017).
- Thompson, M. D. et al. A post glycosylphosphatidylinositol (GPI) attachment to proteins, type 2 (PGAP2) variant identified in Mabry syndrome index cases: Molecular genetics of the prototypical inherited GPI disorder. Eur. J. Med. Genet. 63, 103822 (2020).
- Hansen, L. et al. Hypomorphic mutations in PGAP2, encoding a GPI-anchor-remodeling protein, cause autosomal-recessive intellectual disability. Am. J. Hum. Genet. 92, 575–583 (2013).
- Naseer, M. I. et al. A novel mutation in PGAP2 gene causes developmental delay, intellectual disability, epilepsy and microcephaly in consanguineous Saudi family. J. Neurol. Sci. 371, 121–125 (2016).
- Jezela-Stanek, A. et al. Congenital disorder of glycosylphosphatidylinositol (GPI)-anchor biosynthesis—The phenotype of two patients with novel mutations in the PIGN and PGAP2 genes. Eur. J. Paediatr. Neurol. 20, 462–473 (2016).
- Krawitz, P. M. et al. PGAP2 mutations, affecting the GPI-anchor-synthesis pathway, cause hyperphosphatasia with mental retardation syndrome. Am. J. Hum. Genet. 92, 584–589 (2013).
- Maeda, Y. & Kinoshita, T. Structural remodeling, trafficking and functions of glycosylphosphatidylinositol-anchored proteins. Prog. Lipid Res. 50, 411–424 (2011).
- Tashima, Y. et al. PGAP2 is essential for correct processing and stable expression of GPI-anchored proteins. Mol. Biol. Cell 17, 1410–1420 (2006).
- Lukacs, M., Roberts, T., Chatuverdi, P. & Stottmann, R. W. Glycosylphosphatidylinositol biosynthesis and remodeling are required for neural tube closure, heart development, and cranial neural crest cell survival. Elife 8, (2019).
- Kinoshita, T. Biosynthesis and biology of mammalian GPI-anchored proteins. Open Biology vol. 10 (2020).
- Liu, Y. S. & Fujita, M. Mammalian GPI-anchor modifications and the enzymes involved. Biochemical Society Transactions vol. 48 1129–1138 (2020).
- Kinoshita, T. & Fujita, M. Biosynthesis of GPI-anchored proteins: Special emphasis on GPI lipid remodeling. Journal of Lipid Research vol. 57 6–24 (2016).
- Ghugtyal, V., Vionnet, C., Roubaty, C. & Conzelmann, A. CWH43 is required for the introduction of ceramides into GPI anchors in Saccharomyces cerevisiae. Mol. Microbiol. 65, 1493–1502 (2007).
- Stottmann, R. W. et al. Focusing forward genetics: a tripartite ENU screen for neurodevelopmental mutations in the mouse. Genetics 188, 615–624 (2011).
- Liu, S. S. et al. A knockout cell library of GPI biosynthetic genes for functional studies of GPI-anchored proteins. Commun. Biol. 4, (2021).
Show More