Lay Summary
GNE-CDG, also commonly called GNE myopathy, is a rare inherited condition that causes progressive weakening of muscles (myopathy), starting in adulthood. GNE-CDG is a common congenital disorder of glycosylation (CDG), with over 1000 cases reported to date. GNE-CDG is classified as a disorder of multiple glycosylation pathways and is more specifically a disorder of monosaccharide synthesis. GNE-CDG is caused when an individual has mutations in both copies of their GNE gene, which provides instructions for making a protein that performs the first two steps in the production of sialic acid, a sugar (monosaccharide) that is often attached to the ends of sugar chains of glycosylated proteins and lipids. Unlike most CDG, symptoms of GNE-CDG do not begin until early adulthood, and the primary symptom is a progressive weaking of muscles that may happen over decades. Muscle biopsy may show some abnormalities, but a definitive diagnosis is only achieved through genetic testing; other tests commonly used for CDG diagnosis are not useful. There are currently no approved treatments for GNE-CDG, but several therapies are being explored, with one in a human clinical trial.
Overview
Glucosamine (UDP-N-Acetyl)-2-epimerase/N-Acetylmannosamine kinase congenital disorder of glycosylation (GNE-CDG) is a rare genetic disorder that can present as two distinct syndromes1–3. The more common presentation involves underproduction of the sialic acid N-acetylneuraminic acid (Neu5Ac), a sugar that is incorporated onto the ends of many glycan chains and causes progressive weakening of muscles (myopathy), starting in early adulthood. The less common presentation involves overproduction of Neu5Ac and excess excretion of sialic acid in the urine (sialuria), which causes multisystemic illness that starts at birth but may see significant improvement in adulthood. This page is focused on the more common presentation of GNE-CDG involving myopathy.
The GNE gene encodes GNE, a bifunctional protein that performs the first two steps in the synthesis of Neu5Ac in the cytoplasm from the nucleotide sugar UDP-GlcNAc (Figure 1), making N-acetylmannose-6-phosphate (ManNAc-6-P). Neu5Ac is the predominant sialic acid in human cells and is an important sugar for the glycan component of many N-glycoproteins, O-glycoproteins, and glycolipids; Neu5Ac can also be converted to other important sialic acids4. Deficiency in GNE reduces the amount of ManNAc-6-P being made, leading to less sialic acid and under-sialylation (hyposialylation) of muscle glycans, ultimately resulting in progressive weaking of the muscles.
The first cases of GNE-CDG (myopathy) were reported in the early 1980s as separate syndromes, “distal myopathy with rimmed vacuoles (DMRV)” or “hereditary inclusion body myopathy”5,6. Mutations in the GNE gene were identified in both disorders in 2001 and 2002, reconciling them as the same disease, now called GNE myopathy or GNE-CDG1,7,8. The prevalence of GNE myopathy is estimated at 1 to 9 patients per million people, and over 1,000 individuals with GNE myopathy have been reported9. Symptoms begin between age 20 and 40 and typically start as weakness in the lower leg muscles (tibialis anterior), and progressive muscle weakening may occur over the following decades, with characteristic sparing of quadricep strength until much later in the disease course. Definitive diagnosis can only be achieved through genetic testing, and results from standard tests used in CDG diagnosis (e.g. transferrin and apolipoprotein CIII analysis) are unremarkable2. Currently, there is no cure or approved treatment for GNE-CDG and therapy is based on management of symptoms, but several treatments are being actively explored.
Synonyms
- GNE myopathy (GNEM)
- Distal myopathy with rimmed vacuoles (DMRV)
- Nonaka myopathy
- Vacuolar myopathy sparing the quadriceps
- Quadriceps-sparing myopathy
- Hereditary inclusion myopathy (HIBM)
- Inclusion body myopathy 2
- UDP-GlcNAc epimerase-kinase deficiency
Inheritance
GNE-CDG is an autosomal recessive disorder, meaning an affected individual inherits one defective copy of the gene from each asymptomatic parent.
Gene Function
The GNE gene encodes the enzyme glucosamine (UDP-N-Acetyl)-2-epimerase/N-Acetylmannosamine kinase (GNE). GNE is bifunctional, with two distinct catalytic domains – an epimerase and a kinase domain. It catalyzes the first two steps in the conversion of UDP-GlcNAc to the most common sialic acid, Neu5Ac.
Sialic Acid Synthesis
The synthesis of sialic acid occurs in the cytoplasm. The first, rate-limiting step of this process involves the epimerase domain of GNE and converts the activated sugar UDP-GlcNAc to N-acetylmannose (ManNAc). The second step involves the kinase domain and converts N-acetylmannose to N-acetylmannose-6-phosphate (ManNAc-6-P). Two additional steps, carried about by different enzymes, convert ManNAc-6-P to Neu5Ac (Figure 1).
Once Neu5Ac is synthesized, it is converted into the activated sugar CMP-sialic acid by CMP-sialic acid synthase in the nucleus. CMP-sialic acid is then imported into the Golgi by the CMP-sialic acid transporter SLC35A1. In the Golgi, sialyltransferases facilitate the transfer of sialic acid from CMP-sialic acid onto the terminal ends of N-glycans, O-glycans, glycosphingolipids, or GPI anchor side chains10. Thus, reduced levels of Neu5Ac that result from inadequate GNE activity can affect all major glycosylation pathways.
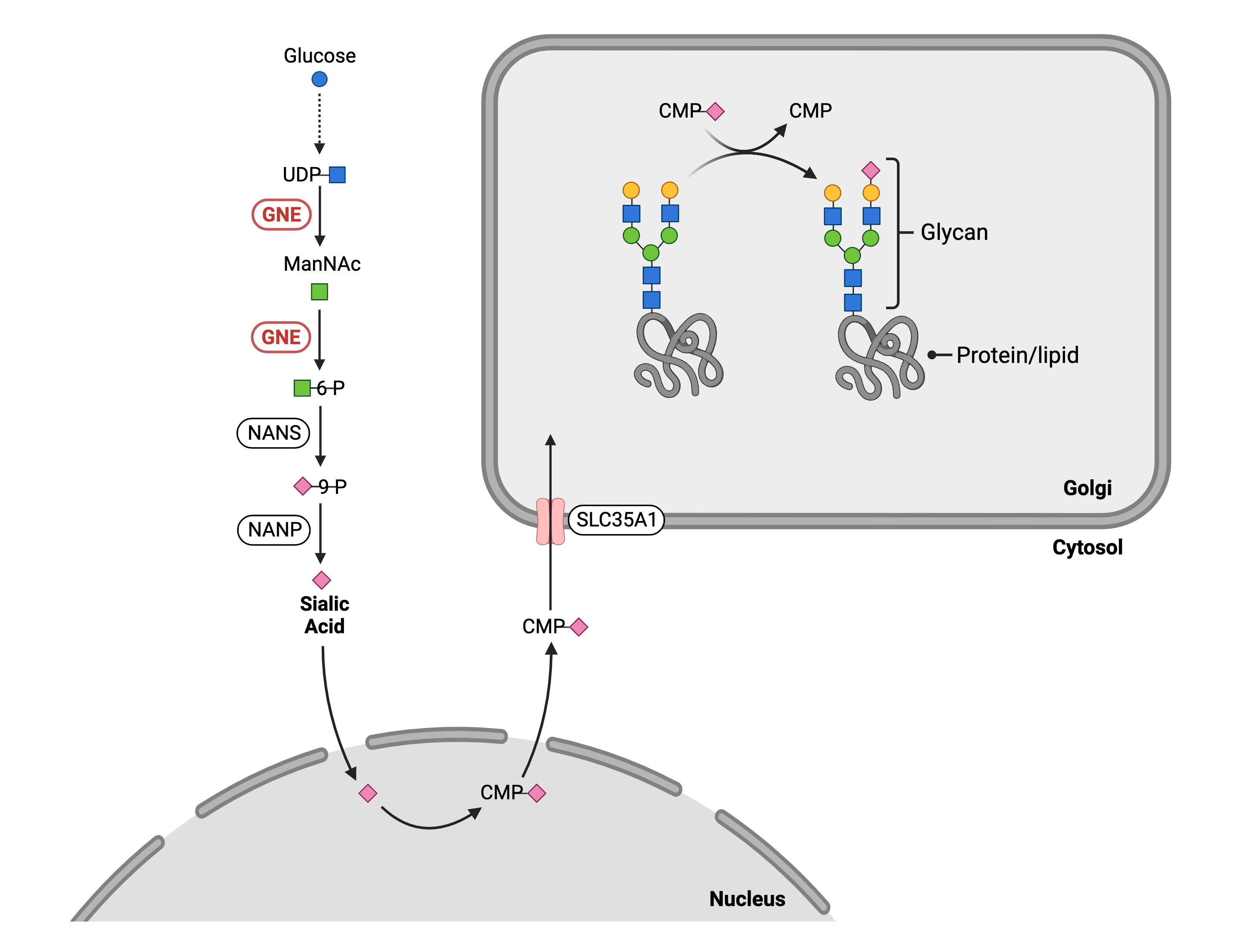
Figure 1. Role of GNE in glycosylation.
GNE is an enzyme that has two roles in glycosylation: 1) GNE converts UDP-GlcNAc to ManNAc, and 2) ManNAc to mannose-6-phosphate. GNE is needed for cells to generate the activated form of sialic acid (CMP-sialic acid) which is attached to glycans in the Golgi.
Disease Mechanism
Mutations in the GNE gene lead to the production of an abnormal enzyme with a 30-60% decrease in activity in either the isomerase or kinase domains, resulting in less formation of ManNAc-6-P and ultimately less Neu5Ac and downstream sialic acid production. Although the exact pathway leading to disease remains unknown, hyposialylation of muscle glycans is believed to contribute to the disease, as sialylation of glycans in other tissues appear2,11. Other mechanisms may also contribute to disease progression. For example, GNE forms a complex with alpha-actinin 2, a structural protein that is important for muscle cell stability and contraction, and this interaction is reduced 10-fold when the GNE has the most frequent mutation observed in GNE myopathy12. As well, decreased levels of sialic acid can lead to oxidative stress in the ER, resulting in muscle cell atrophy13,14.
Mutations
The GNE gene is found on Chromosome 9 (9p13.3) and over 255 mutations, almost entirely missense, have been identified resulting in between 30-60% decrease in enzyme activity9,15. Phenotype/genotype relationships remain elusive, and mutations in GNE are only able to predict 20% of disease severity16. Mutations that cause total loss of enzyme activity appear incompatible with life.
Signs & Symptoms
Clinical Presentation
Individuals with GNE-CDG typically develop symptoms in between the ages of 20 and 40, starting with difficulty lifting the front part of the foot (foot drop). The myopathy progresses slowly over the course of the patient’s life.
- Muscle – progressive weakening of leg muscles, sparing the quadriceps until later in the disease. Most patients require a wheelchair 10 to 20 years after the onset of symptoms. Eventually all skeletal muscles may be impacted
- Breathing – some patients may require breathing support in late-stage disease due to weakening of respiratory muscles
Biochemical Abnormalties
Apart from a modest increase in serum creatine kinase (2-5x normal)9, GNE-CDG does not have significant biochemical abnormalities.
Diagnosis
GNE myopathy should be suspected in any slowly progressing myopathy that starts with weakness in the lower extremities. Diagnostic tests used to diagnose other CDG (e.g. serum transferrin and apolipoprotein CIII analysis) are unremarkable in GNE myopathy. Biopsy of impacted muscles typically shows rimmed vacuoles, variation of muscle fiber size, atrophy, and no inflammation. Some biomarkers may be used to help identify GNE myopathy, but direct molecular genetic testing is the only way to definitively diagnose the disorder.
Biomarkers
GNE myopathy impacts sialylation of several antigens. GNE myopathy patients may have increased serum levels of desialylated Thomsen-Friedenreich antigen (T) and/or decreased levels of the sialylated form, ST; the ratio of T/ST was found to be higher in all tested GNE myopathy patients when compared to controls17. As well, the level of Krebs von den Lungen 6 (KL-6) antigen is increased in the serum of GNE myopathy patients18.
Classification
GNE-CDG is a disorder of multiple glycosylation pathways, and more specifically a disorder of monosaccharide synthesis.
Prognosis
Life span is not thought to be impact by GNE-CDG, but patients can lose control of skeletal muscles and become dependent on caregivers9.
Management
Management of GNE-CDG requires a multidisciplinary team and may include combinations of physical therapy, occupational therapy, and support from a pulmonologist. Individuals with GNE myopathy should pursue a healthy diet and exercise but avoid lifting weights and activities that cause muscle pain9.
Therapies
There are currently no treatment options available for GNE-CDG, but several therapies have been explored or are in development. The goal of most therapies is to increase the amount of sialic acid available for use in the cells by providing substrates that can be metabolized into sialic acid. Several gene-based therapies have been explored, with the goal of providing working copies of the GNE gene in muscle cells.
Sialic Acid Supplementation
Oral sialic acid supplementation, in both rapid and extended-release formats, has been extensively explored for the treatment of GNE myopathy in humans. Early studies show that it was safe and well tolerated, but a large well-designed study conclusively demonstrated that oral sialic acid administration was not better than placebo at improving muscle strength in GNE patients2,19,20. This is likely because sialic acid is not efficiently absorbed into cells for use in the Golgi. A synthetic derivative of sialic acid, sialyllactose, improves GNE myopathy symptoms in mice, but has not been studied in humans21.
ManNAc Supplementation and ManNAc Derivatives
The rate limiting step of sialic acid synthesis is the conversion of UDP-GlcNAc to ManNAc by the epimerase domain of GNE. It was theorized that oral supplementation of ManNAc would increase the amount of ManNAc available for use in the synthesis of sialic acid in cells. Results obtained in mouse models and initial clinical trials appear promising, and this strategy is now being studied in a large, placebo-controlled, double-blind, Phase 2 clinical trial11,22–24.
Although the studies show that ManNAc supplementation is safe and well-tolerated, the therapy requires daily large doses of ManNAc. Other work has pursued synthetic derivatives of ManNAc that could be used at lower doses. For example, peracetylated ManNAc is better able to enter cells before being converted to ManNAc and has shown promising improvement of muscle phenotype in GNE myopathy mice but has not been explored in humans25. As well, a derivative of ManNAc-6-P, which is the product of the kinase domain of GNE, was more effective at restoring sialic acid levels than ManNAc in GNE deficient cell lines but has not been explored in animal models26.
IVIG Administration
Immunoglobin (IG) is a glycoprotein with multiple sialic acid residues present on its glycan chains. Intravenous (IV) administration of IG results in its uptake in cells and subsequent breakdown, releasing sialic acid. This strategy was explored in four GNE myopathy patients and showed mild improvement in the strength of some muscles but not change to muscle glycan sialylation27,28. IVIG has not been further pursued, possibly because it requires large weekly doses of the drug delivered by IV.
Gene Therapies
The goal of gene therapy is to deliver functional copies of the GNE gene to muscle cells so that they can produce functional GNE, or to directly edit and correct the errors in the mRNA produced from the mutated gene. These therapies have been used to produce functional GNE in cells derived from GNE myopathy patients and mouse models of GNE29–33. As well, a human GNE myopathy patient received intramuscular (IM) and intravenous (IV) administration of a plasmid-based gene GNE therapy31,32. These therapies were well-tolerated and wildtype GNE expression was confirmed after both methods of administration of the plasmid, but overall improvement in the patient was limited, possibly reflecting difficulties in treating the later stages of the disease using this methodology. Larger clinical studies are needed to confirm safety and efficacy of gene therapies in treating GNE myopathy.
Research Models
Several GNE research models have been generated including zebrafish and mouse models, and human cell lines.
Fish (D. rerio)
Gne morpholino knockdown
The Zebrafish gne gene is highly homologous to the human ortholog GNE, and was targeted to make a morpholino knockdown model of GNE myopathy39. Morphant embryos had significantly reduced locomotor activity, distorted muscle integrity, and a reduction in muscle fibers.
Mouse (M. musculus)
Gne-/- constitutive knockout
Gne is orthologous to human GNE, and Gne knock-out is embryonic lethal between 8.5-9.5 days post coitum34.
GNE-deficient transgenic mice (Gne-/-hGNED176V-Tg)
A transgenic mouse model wherein mice do not possess the Gne gene but are instead homozygous for the human GNED176V allele; the D176V mutation is one of the most prevalent mutations in Japanese GNE myopathy patients35. These Gne(-/-)hGNED176V-Tg mice demonstrate decreased levels of sialic acid, particularly in the serum. They also have lower survival rates and decreased muscle strength and motor starting after 30 weeks compared to heterozygous littermates. Histological similarities to GNE myopathy are present in muscle fibers, and rimmed vacuoles are formed at 42 weeks.
Homozygous GneM712T/M712T
This genotype corresponds to a GNE founder mutation in Persian-Jewish GNE myopathy patients. Unexpectedly, this mutation leads to severe glomerular proteinuria and hematuria, resulting in kidney failure and death within 3 days after birth36. Feeding females ManNAc during matings and pregnancy increased survival past 3 days in 43% of mutant offspring mice, and similar rescue of kidney sialylation and pathology was noted when pregnant female mice were fed mannose36,37. This mutation was bred into several strains of mice, and the GneM712/M712T F2 generation mice displayed three distinct phenotypes: severe (38% died within 7 days after birth), moderate (54% died between weeks 2-13), and normal (8% of survived more than 36 weeks)38. Further crossing of GneM712/M712T mice with no apparent phenotype led to GneM712/M712T mice with high and long-term survival rates.
Models for Gene Therapy
Healthy mice have been used to explore the sustained expression and safety of wildtype human GNE after gene administration through either adeno associated virus type 8 (AAV8) systemic delivery or intramuscular and systemic injection of GNE plasmid lipoplexes31,33.
Human Cell Lines
GNE myopathy-derived muscle cells
Transduction of patient-derived muscle cells with normal GNE via AAV8 vector resulted sustained expression of GNE33.
Clinical Studies
Active
Multi-Center Study of MacNAc for GNE Myopathy (MAGiNE) (NCT04231266)
Leadiant Biosciences, Inc., in collaboration with Brigham and Women’s Hospital, the National Human Genome Research Institute, National Institute of Arthritis and Musculoskeletal and Skin Diseases, and the National Institute of Neurological Disorders and Stroke, is conducting a randomized, placebo-controlled, double-blind Phase 2 trial on the long-term safety and efficacy of oral ManNAc supplementation to treat GNE myopathy. This builds off the safety and efficacy of ManNAc supplementation demonstrated in previous trials (read below).
International GNE Myopathy Patient Registry (NCT04009226)
Newcastle University has created an online patient registry platform to longitudinally collect information from GNE myopathy patients. The information will allow a better understanding of long-term disease course and provide a database of patients who may be eligible for future clinical research.
A Natural History Study of Patients With GNE Myopathy and GNE-Related Diseases (NCT01417533)
National Human Genome Research Institute, in collaboration with Therapeutics for Rare and Neglected Diseases, is conducting a natural history study on patients with GNE myopathy. Patient disease progression and other health parameters will be monitored over a 2-year period to better understand causes, symptoms, and effects of GNE myopathy. Results from this study have been used to determine how MRI can be used to noninvasively determine disease severity in GNE myopathy40.
Clinical and Basic Investigations into Congenital Disorders of Glycosylation (NCT04199000)
The Frontiers in Congenital Disorder of Glycosylation Disorders Consortium (FCDGC) is conducting a 5-year natural history study on all CDG types, including GNE-CDG. The purpose of this study is to define the natural history and clinical symptoms of CDG, develop new diagnostic techniques, identify clinical biomarkers that can be used in future clinical trials and evaluate whether dietary treatments improve clinical symptoms and quality of life.
Completed
An Open Label Phase 2 Study of ManNAc in Subjects With GNE Myopathy (NCT02346461)
The National Human Genome Research Institute, in collaboration with the National Center for Advancing Translational Science, completed a Phase 2 on the use of ManNAc to treat GNE myopathy by increasing the amount of Neu5Ac present in the plasma and improving sialylation of muscle glycans22. The trial demonstrated long-term safety and clinical efficacy, as determined by a slower rate of decline in muscle strength but was limited by its small sample size. The study was also used to help evaluate ManNAc dosing regimens to optimize Neu5Ac production23.
Phase I Clinical Trial of ManNAc in Patients with GNE Myopathy or Hereditary Inclusion Body Myopathy (HIBM) (NCT01634750)
The National Human Genome Research Institute, in collaboration with Therapeutics for Rare and Neglected Diseases and the National Center for Advancing Translational Science, conducted a Phase 1 trial that determined that oral ManNAc supplementation was well tolerated in people with GNE myopathy. The study was also used to help evaluate ManNAc dosing regimens to optimize Neu5Ac production23.
GNE-Myopathy Disease Monitoring Program (GNEM-DMP): A Registry and Prospective Observational Natural History Study to Assess GNE Myopathy or Hereditary Inclusion Body Myopathy (HIBM) (NCT01784679)
Ultragenyc Pharmaceutical Inc., in collaboration with Newcastle University, conducted a natural history study on patients with GNE myopathy. Patient disease progression and other health parameters were monitored to better understand causes, symptoms, and biomarkers of GNE myopathy.
Phase 3 Randomized, Double-Blind, Placebo-Controlled Study to Evaluate Sialic Acid in Patients With Glucosamine (UDP-N-acetyl)-2-epimerase Myopathy (GNEM) or Hereditary Inclusion Body Myopathy (HIBM) (GNEM) (NCT02377921)
Building off promising data on the safety and efficacy of oral sialic acid administration for treating GNE myopathy (NCT01236898, NCT04671472), Ultragenyx licensed rights to extended release sialic acid (Ace-ER) from Nobelpharma, AAIPharm, and the HIBM Research Group and pursued further clinical trials using the drug (NCT02736188, NCT02731690, NCT01359319, NCT01830972, NCT01517880). This culminated in a Phase 3, double-blind, placebo-controlled study in 89 GNE myopathy patients which provided Class 1 evidence demonstrating that oral sialic acid supplementation does not improve muscle strength in GNE myopathy19.
Intravenous Immune Globulin to Treat Hereditary Inclusion Body Myopathy (NCT00195637)
National Human Genome Research Institute conducted a Phase 1 clinical trial that explored the effect of administering immune globin (IG), a glycoprotein with multiple sialic acid residues, via IV to four patients with GNE myopathy over a one-month period. The goal of the study was to determine if the sialic acid in IG would be absorbed by muscles cells and improve muscle function. Muscle strength seemed to improve during treatment, with improvements lost within two weeks of stopping treatment, but the study was limited by its short time frame and limited number of participants41.
Retrospective Cohort Study Assessing the Natural Course in Congenital Cataract Facial Dysmorphism Neuropathy Syndrome (CCFDN) and Sporadic and Hereditary Inclusion Body Myopathies (IBM) (NCT01902940)
The Ludwig-Maximilians – University of Munich conducted a retrospective study on 350 patients with either CCFDN or GNE myopathy to better understand the natural course of the disease.
Clinical, Biological and NMR Outcome Measures Study for Hereditary Inclusion Body Myopathy Due to Mutation of UDP-N-acetylglucosamine 2-epimerase/N-acetylmannosamine Kinase Gene (GNE) (ClinBio-GNE) (NCT02196909)
The Institut de Myologie (France) conducted a longitudinal study on 20 GNE myopathy patients to identify clinical and biological outcome measures that might be useful in future clinical trials.
Organizations
Publications
GNE-CDG Scientific Articles on PubMed
Additional Resources
GeneReviews
OMIM
OrphaNet
GARD
Genetic Testing Registry
ClinVar
NIH
GeneCards
UniProt
References
- Eisenberg, I. et al. The UDP-N-acetylglucosamine 2-epimerase/N-acetylmannosamine kinase gene is mutated in recessive hereditary inclusion body myopathy. Nat. Genet. 29, 83–87 (2001).
- Carrillo, N., Malicdan, M. C. & Huizing, M. GNE Myopathy: Etiology, Diagnosis, and Therapeutic Challenges. Neurotherapeutics 15, 900–914 (2018).
- Awasthi, K., Srivastava, A., Bhattacharya, S. & Bhattacharya, A. Tissue specific expression of sialic acid metabolic pathway: role in GNE myopathy. J. Muscle Res. Cell Motil. 42, 99–116 (2021).
- Varki, A. Diversity in the sialic acids. Glycobiology 2, 25–40 (1992).
- Nonaka, I., Sunohara, N., Satoyoshi, E., Teresawa, K. & Yonemoto, K. Autosomal recessive distal muscular dystrophy: A comparative study with distal myopathy with rimmed vacoule formation. Ann. Neurol. 17, 51–59 (1985).
- Nonaka, I., Sunohara, N., Ishiura, S. & Satoyoshi, E. Familial distal myopathy with rimmed vacuole and lamellar (myeloid) body formation. J. Neurol. Sci. 51, 141–155 (1981).
- Nishino, I. et al. Distal myopathy with rimmed vacuoles is allelic to hereditary inclusion body myopathy. Neurology 59, 1689–1693 (2002).
- Noguchi, S. et al. Reduction of UDP-N-acetylglucosamine 2-Epimerase/N-Acetylmannosamine Kinase Activity and Sialylation in Distal Myopathy with Rimmed Vacuoles. J. Biol. Chem. 279, 11402–11407 (2004).
- Carrillo, N., Malicdan, M. C. & Huizing, M. GNE Myopathy. in GeneReviews® [Internet] (eds. Adam, M. P., Ardinger, H. H. & Pagon, R. A. et al.) (University of Washington, Seattle, 2020).
- Varki, A. & Schauer, R. Sialic Acids. in Essentials of Glycobiology (eds. Varki, A., Cummings, R. D. & Esko, J. D. et al.) 335–349 (Cold Spring Harbor Laboratory Press, 2009). doi:10.1007/978-3-540-76698-8_19.
- Xu, X. et al. Safety, pharmacokinetics and sialic acid production after oral administration of N-acetylmannosamine (ManNAc) to subjects with GNE myopathy. Mol. Genet. Metab. 122, 126–134 (2017).
- Harazi, A. et al. The Interaction of UDP-N-Acetylglucosamine 2-Epimerase/N-Acetylmannosamine Kinase (GNE) and Alpha-Actinin 2 Is Altered in GNE Myopathy M743T Mutant. Mol. Neurobiol. 54, 2928–2938 (2017).
- Chanana, P., Padhy, G., Bhargava, K. & Arya, R. Mutation in GNE Downregulates Peroxiredoxin IV Altering ER Redox Homeostasis. NeuroMolecular Med. 19, 525–540 (2017).
- Cho, A. et al. Sialic acid deficiency is associated with oxidative stress leading to muscle atrophy and weakness in GNE myopathy. Hum. Mol. Genet. 26, 3081–3093 (2017).
- Argov, Z. GNE myopathy: a personal trip from bedside observation to therapeutic trials. Acta Myol. 33, 107–110 (2014).
- Pogoryelova, O. et al. GNE genotype explains 20% of phenotypic variability in GNE myopathy. Neurol. Genet. 5, (2019).
- Leoyklang, P. et al. Sialylation of Thomsen-Friedenreich antigen is a noninvasive blood-based biomarker for GNE myopathy. Biomark. Med. 8, 641–652 (2014).
- Kurashige, T., Takahashi, T., Nagano, Y., Sugie, K. & Maruyama, H. Krebs von den Lungen 6 decreased in the serum and muscle of GNE myopathy patients. Neuropathology 41, 29–36 (2021).
- Lochmüller, H. et al. A phase 3 randomized study evaluating sialic acid extended-release for GNE myopathy. Neurology 92, E2109–E2117 (2019).
- Pogoryelova, O., González Coraspe, J. A., Nikolenko, N., Lochmüller, H. & Roos, A. GNE myopathy: From clinics and genetics to pathology and research strategies. Orphanet J. Rare Dis. 13, (2018).
- Yonekawa, T. et al. Sialyllactose ameliorates myopathic phenotypes in symptomatic GNE myopathy model mice. Brain 137, 2670–2679 (2014).
- Carrillo, N. et al. Safety and efficacy of N-acetylmannosamine (ManNAc) in patients with GNE myopathy: an open-label phase 2 study. Genet. Med. 23, 2067–2075 (2021).
- Van Wart, S., Mager, D. E., Bednasz, C. J., Huizing, M. & Carrillo, N. Population Pharmacokinetic Model of N-acetylmannosamine (ManNAc) and N-acetylneuraminic acid (Neu5Ac) in Subjects with GNE Myopathy. Drugs R D 21, 189–202 (2021).
- Malicdan, M. C. V, Noguchi, S., Hayashi, Y. K., Nonaka, I. & Nishino, I. Prophylactic treatment with sialic acid metabolites precludes the development of the myopathic phenotype in the DMRV-hIBM mouse model. Nat. Med. 15, 690–696 (2009).
- Malicdan, M. C. V. et al. Peracetylated N-acetylmannosamine, a synthetic sugar molecule, efficiently rescues muscle phenotype and biochemical defects in mouse model of sialic acid-deficient myopathy. J. Biol. Chem. 287, 2689–2705 (2012).
- Morozzi, C. et al. Targeting GNE Myopathy: A Dual Prodrug Approach for the Delivery of N-Acetylmannosamine 6-Phosphate. J. Med. Chem. 62, (2019).
- Nishino, I., Carrillo-Carrasco, N. & Argov, Z. GNE myopathy: current update and future therapy. J. Neurol. Neurosurg. Psychiatry 86, 385–392 (2015).
- Sparks, S. et al. Intravenous immune globulin in hereditary inclusion body myopathy: A pilot study. BMC Neurol. 7, 1–13 (2007).
- Tal-Goldberg, T., Lorain, S. & Mitrani-Rosenbaum, S. Correction of the Middle Eastern M712T mutation causing GNE myopathy by trans-splicing. NeuroMolecular Med. 16, 322–331 (2014).
- Nemunaitis, G. et al. Hereditary inclusion body myopathy: Single patient response to intravenous dosing of GNE gene lipoplex. Hum. Gene Ther. 22, 1331–1341 (2011).
- Phadke, A. P. et al. Safety and in vivo Expression of a GNE-Transgene: A Novel Treatment Approach for Hereditary Inclusion Body Myopathy-2. Gene Regul. Syst. Bio. 3–89 (2009).
- Nemunaitis, G. et al. Hereditary inclusion body myopathy: single patient response to GNE gene Lipoplex therapy. J. Gene Med. 12, 403–412 (2010).
- Mitrani-Rosenbaum, S. et al. Sustained expression and safety of human GNE in normal mice after gene transfer based on AAV8 systemic delivery. Neuromuscul. Disord. 22, 1015–1024 (2012).
- Schwarzkopf, M. et al. Sialylation is essential for early development in mice. Proc. Natl. Acad. Sci. U. S. A. 99, 5267–5270 (2002).
- Malicdan, M. C. V., Noguchi, S., Nonaka, I., Hayashi, Y. K. & Nishino, I. A Gne knockout mouse expressing human GNE D176V mutation develops features similar to distal myopathy with rimmed vacuoles or hereditary inclusion body myopathy. Hum. Mol. Genet. 16, 2669–2682 (2007).
- Galeano, B. et al. Mutation in the key enzyme of sialic acid biosynthesis causes severe glomerular proteinuria and is rescued by N-acetylmannosamine. J. Clin. Invest. 117, 1585–1594 (2007).
- Niethamer, T. K. et al. Oral monosaccharide therapies to reverse renal and muscle hyposialylation in a mouse model of GNE myopathy. Mol. Genet. Metab. 107, 748–755 (2012).
- Sela, I. et al. Variable Phenotypes of Knockin Mice Carrying the M712T Gne Mutation. NeuroMolecular Med. 15, 180–191 (2013).
- Daya, A. et al. Gne depletion during zebrafish development impairs skeletal muscle structure and function. Hum. Mol. Genet. 23, 3349–3361 (2014).
- Liu, C. Y. et al. Skeletal Muscle Magnetic Resonance Biomarkers in GNE Myopathy. Neurology 96, e798–e808 (2021).
- Sparks, S. et al. Intravenous immune globulin in hereditary inclusion body myopathy: A pilot study. BMC Neurol. 7, 1–13 (2007).